Improving PFAS remedial groundwater results using mass flux mapping
FluxTracer can now be used on PFAS, not just chlorinated volatile organic compounds
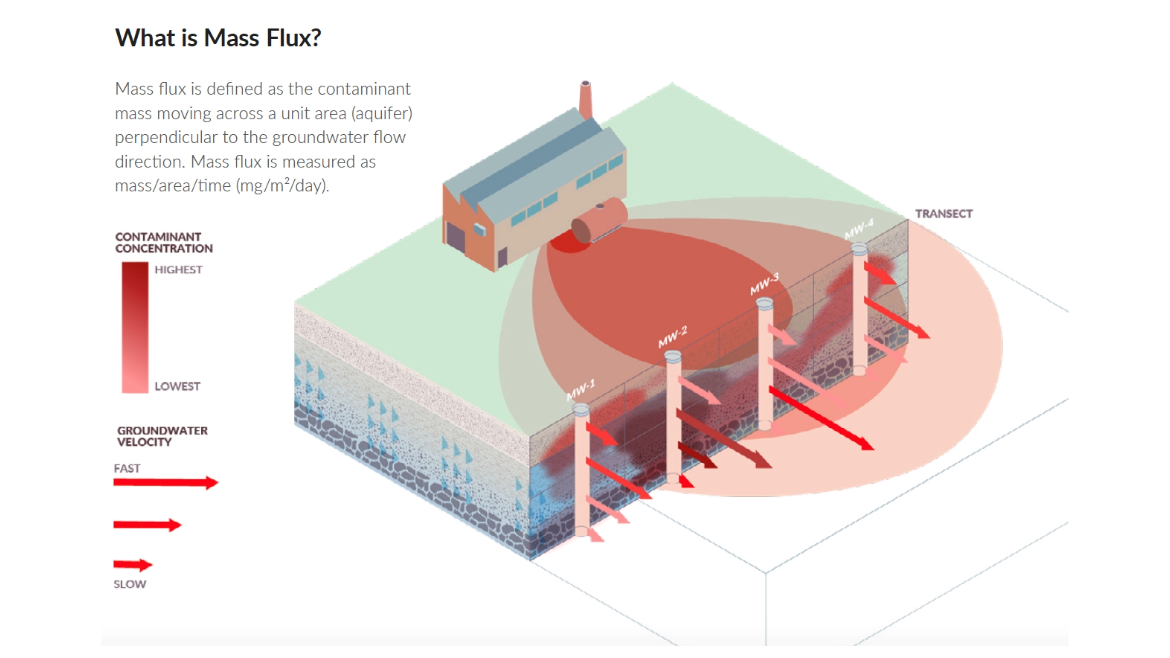
The use of Colloidal Activated Carbon (CAC) technology to remediate PFAS (per- and polyfluoroalkyl substances) is becoming a widely adopted strategy to effectively contain PFAS impacts in situ (i.e., in place) and eliminate the exposure risk upstream of domestic water supply wells, surface waters, and other sensitive receptors. When deploying this remedy, understanding the aquifer zones PFAS contaminants are moving through (i.e., mass flux zones-see Figure 1) to optimize performance efficiency and reduce project costs is of great value to the overall success of the project.
The FluxTracer® Flux Mapping Tool is a flux measuring device used to accurately measure contaminant mass flux—the product of the contaminant concentration and groundwater flux (or Darcy velocity)—before implementing an in situ remedy. Previously available for chlorinated volatile organic compounds (CVOCs), the tool is now available to measure PFAS mass flux using DEXSORB® for PFAS.
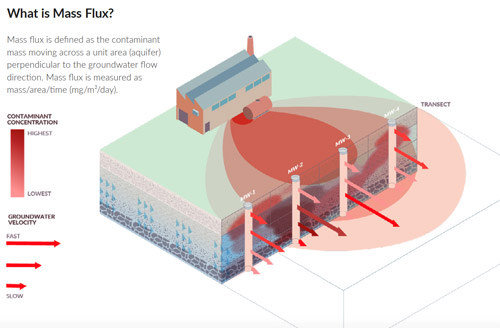
PRB Usage in PFAS Remediation and the Role of Mass Flux
Permeable reactive barriers, or PRBs, are among the most frequently applied remedies for groundwater contamination. They are commonly installed to prevent the migration of PFAS and other contaminants from an impacted site, protecting against downgradient exposure risk.
PRBs using CAC create in situ filtration zones, removing PFAS from the dissolved phase and retaining the contaminants on the aquifer matrix. The in situ PFAS treatment method is quickly gaining adoption globally based on its proven results in the field, a 98 percent lower carbon footprint, and substantially lower costs compared to pump & treat alternatives to achieve the same risk reduction goals. These PRBs employing CAC can be applied at the leading edge of a PFAS plume to prevent further plume migration or at intervals within a plume body to treat large, diffuse groundwater PFAS plumes holistically (Figure 2).
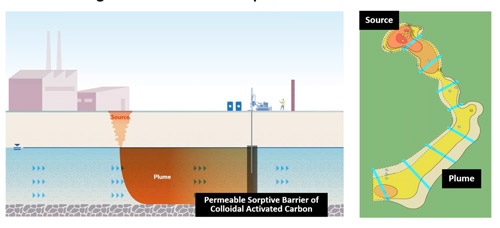
Knowing the mass flux is critical for engineering a successful PRB treatment approach, whether it be for PFAS or any other contaminant class. This parameter determines the placement of amendment(s) needed to achieve the project goals and the dosing needed to attain them at the lowest possible cost. Mass flux is directly correlated to CAC or other amendment consumption, dictating the length of time a remedy will remain effective.
Mass flux is rarely uniform and is instead highly variable in an aquifer. Even within a thin slice of a contaminated aquifer where a PRB is installed, contaminant flux measurements typically span orders of magnitude. Although remedial amendment selection and dosing must account for this variability to achieve the best result, its impact is frequently neglected.
Bulk Aquifer Measurement Methods are Not Appropriate for Estimating Mass Flux in PRBs
Remediation practitioners have historically relied on pumping tests or slug tests to measure hydraulic conductivity and estimate groundwater velocity. While these methods are fundamental for general aquifer characterization and testing/developing water supplies, they are generally not useful for in situ groundwater remediation. These more traditional methods can lead a project astray when used in modeling for in situ groundwater remediation.
Studies have shown most contaminant mass making up a groundwater contaminant plume flows through mass flux zones occupying only a minor fraction (e.g., 5-10%) of the aquifer, even in homogenous sandy aquifers[1]. In contrast, pumping tests and slug tests produce average hydraulic conductivities representing relatively large aquifer volumes. Contaminant concentration data are also averages, sampled from groundwater entering through screens over lengths ranging from 5 to 20 feet.
As a simplified example, consider a PRB placed to treat a contaminant plume moving across the barrier location at an average flux of 20 mg/m2/day flux, calculated from one or two wells over a 10 feet vertical depth (i.e., the length of a typical monitoring well screen). A vastly different PRB design would be required for a mass flux evenly distributed across the barrier to accommodate an average flux, vs. a true flux greater than 100 mg/m2/day but over only 20 percent of the PRB's cross-sectional treatment area (Figure 3).
By mixing and averaging discrete mass flux zones with the bulk of the plume that is not carrying contaminants, the resolution of contaminant flux is lost, and contaminant transport becomes mischaracterized. PRB designs not accounting for this mass flux variance can underdose the high-mass flux zones and place amendments where they are not needed, producing something nobody wants: an overdesigned PRB that doesn't work.
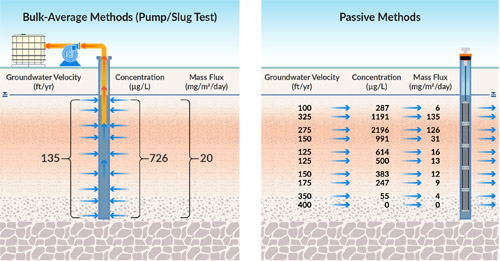
FluxTracer Developed for PFAS
FluxTracer is an easy-to-use passive flux measurement device that vertically delineates groundwater velocity and contaminant flux within existing monitoring wells. The data generated by this device provides remedial designers with important information on the flux zones within the aquifer, which ultimately aids in improving the results of their remediation efforts.
FluxTracer uses sorbent materials spiked with alcohol tracers to identify discrete flux zones (Figure 4). The device is typically deployed in a well for two weeks and then sampled for PFAS at every other foot to provide adequate data resolution for treatment designers. Groundwater flux (i.e., Darcy velocity) is calculated from the tracer loss, and PFAS mass flux is calculated directly from the mass of contaminants that become sorbed during the two-week deployment. Following analysis, the data are presented in a graphical form to aid the remediation design process (Figure 5).
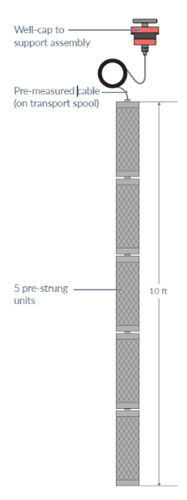
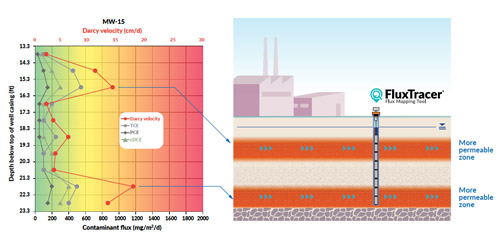
Applying Flux-Informed Remedies at PRBs
The discrete information provided by FluxTracer, incorporating Cyclopure's 1DEXSORB, offers a much more detailed picture of PFAS mass flux across a CAC PRB. With this more detailed picture of the PFAS transport zones controlling plume development and driving exposure risks, in situ PFAS remedies can be better optimized, improving remedial outcomes and reducing project costs.
About DEXSORB
DEXSORB® is a patented technology offered by Cyclopure®. For more information: contact@cyclopure.com.
[1] Guilbeault MA, Parker BL, Cherry JA. Mass and Flux Distributions from DNAPL Zones in Sandy Aquifers. Groundwater. 2005;43(1):70-86. doi:10.1111/j.1745-6584.2005.tb02287.x